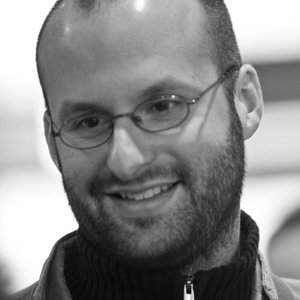
Michael Baudoin
Research activities
ACOUSTOFLUIDICS
An important part of our research activities is dedicated to acoustofluidics, a subject at the interface between acoustics, microfluidics and microsystems. In particular we (i) developed some miniaturized acoustical tweezers enabling the selective and contactless manipulation of microscopic objects and fluids, (ii) worked on the use of SAW to move droplets and clean surfaces and (iii) studied how rapid interfaces reconfiguration can lead to some specific acoustic signatures.
Acoustical tweezers
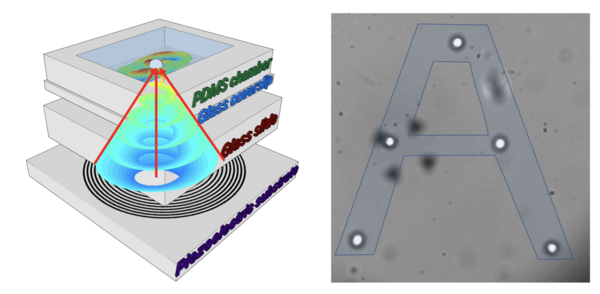
The selective precise remote manipulation of micrometric objects both in vivo and vitro open some outstanding perspectives e.g. in medecine for noninvasive surgery, in micro-robotics for microrobots propulsion or in microbiology for cells and microorganisms contactless manipulation, organization and mechanical properties testing. Our team has designed 2 new types of microtweezers: some holographic single beam tweezers based on spiraling interdigitated transducers enabling the precise selective manipulation and organization of microparticles and cells [A20, A25, A28, A33], and some reconfigurable tweezers based on IDTs arrays and inverse filter enabling real time adative field synthesis [A12, A13, A14].
SAW-based surface cleaning
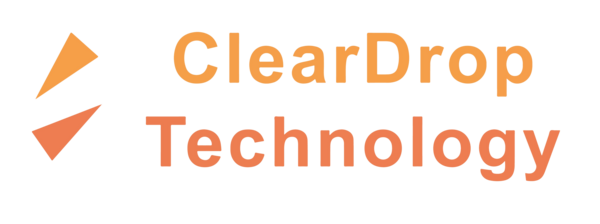
In many applications, the vision can be perturbated by the presence of droplets, snow/ice or dust on optical surfaces. After working for more than ten years on the physics of droplets displacement induced by Surface Acoustic Waves (SAWs) [A3, A7, A35, A39], our team recently funded a startup commericalizing an integrated solution (Cleardrop technology) based on ultrasonic SAWs to clean optical windows. Indeed, these "nano-earthquakes" propagating at the surface of the subtrate are adsorbed by the water droplets lying on the surface provoking their displacement through two nonlinear effects called the acoustic radiation force and acoustic streaming. Note that the droplet displacement is promoted by some low frequency (in the 100Hz range) vibrations of the drop free surface, whose origin was elucidated recently in ref [A39].
Nonlinear acoustics theory
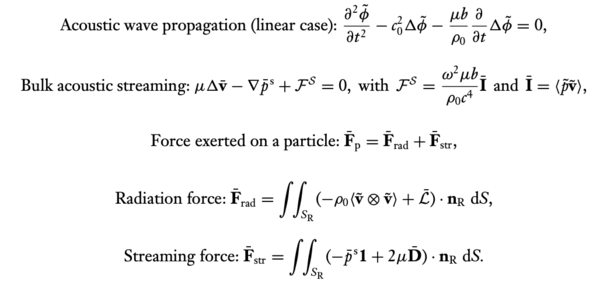
Besides experimental developments, our team has worked on nonlinear acoustics theory and in particular on the acoustic radiation force [A9, A19] and acoutic streaming [A30, A32, A34]. On acoustic streaming, our work include a study of the acoustic streaming produced by acoustical vortices [A9] and a simplified formulation of the source term for the numerical simulation of acoustic streaming [A19] with reduced computational cost. On the acoustic radiation force, our work include (i) an angular spectrum based formulation of the acoustic radiation torque [A30], (ii) an extension to the unsteady regime [A32] of Gork'ov's formula and (iii) a unification of different formulation of the acoustic radiation forcea and torque exerted on a particle by an arbitrary incident wavefield [A34].
INTERFACIAL FLUID MECHANICS
Another part of our research is dedicated to fluid mechanics in the presence of interfaces between two fluids and in particular gas/liquid interfaces with surface tension effects.
Particle covered interfaces
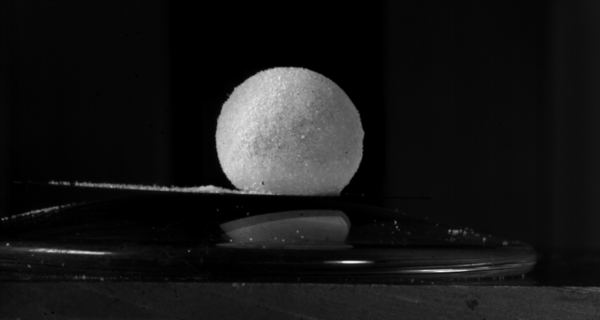
The presence of partially wetting microparticles at the interface between two fluids can drastically modify the mechanical properties of these interfaces and lead to some unexpected behaviors. First, we demonstrated that pushing a liquid finger inside a tube covered with particles enables the tailored synthesis of cylindrical bubbles encapsulated in a monolayer of particles (so-called “armoured bubbles”) [A11, A21], which remain stable even when extracted from the tube. Second, we revisited the Saffman-Taylor instability when particles are adsorbed by the interface between the two fluids [A18]. Finally, we demonstrated that ultra-long lasting (> 1 year) air bubbles resisting drainage, evaporation and nuclei induced bursting can be simply synthesized by replacing surfactants by microparticles and water by a hygroscopic liquid [A36].
Liquid plugs dynamics
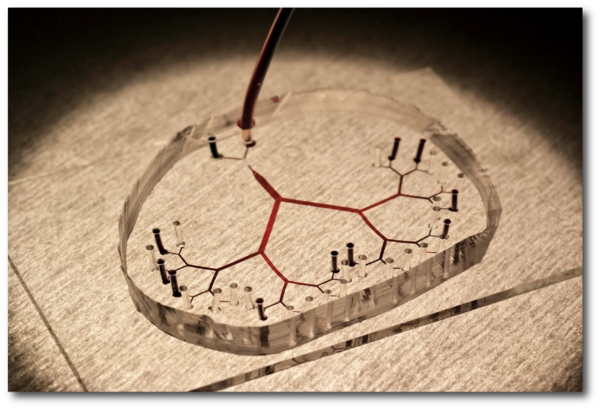
Liquid plugs play a fundamental role both in chemical engineering processes and in medecine where they can form naturally in the lung due to the instability of mucus lining of patients suffering from cystic fibrosis or pulmonary obstructive diseases. Our team has studied the dynamics and rupture of these liquid plug in simplified geometries. Our work include experimental observation and theoretical modeling of cascades of plug ruptures in simple lining and microfluidic tree structures [A8, A17] mimicking the last generations of the lung tree, as well as response of liquid plugs to cyclic forcing [A22,A23]. More recently we showed that pushing a liquid plug at high speed in a cylindrical tube leads to the formation of a long droplet surrounded by a thin air film, a reverse situation compared to Bretherton bubbles [A29].
Sound of interfacial flows
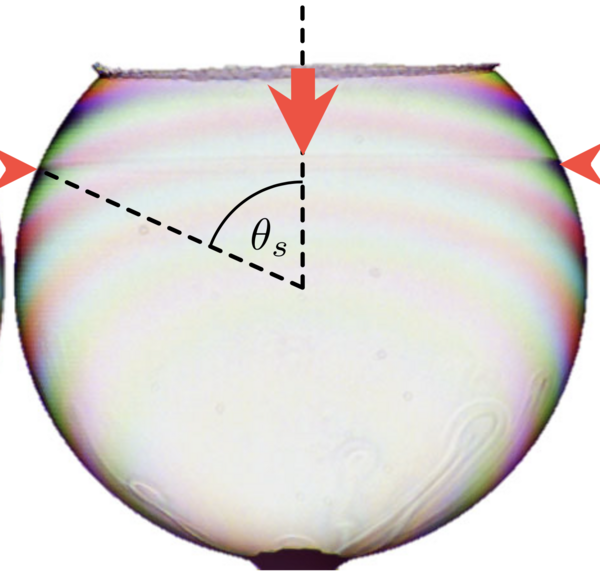
Brutal interfacial reconfigurations of fluid interfaces such as the bursting of a soap bubble [A27] can lead to the emission of a characteristic sound. This sound caries out some information on the underlynig physics of this event. In [A27] we were able to model the popping sound emitted by a bursting bubble using the framework of aeroacoustics, and to show this acoustic emission originates mainly from the capillary stresses exerted by the liquid soap film on the air. This work constitutes a proof of concept that the acoustic signature of violent events of physical or biological origin could be used to measure the forces at play during these events.
QUANTUM ANALOGUES
More recently, we have been working on analogies between Acoustics and Quantum Physics on one hand and Hydrodynamics & Cosmology on the other hand.
Acoustic quantum analogues
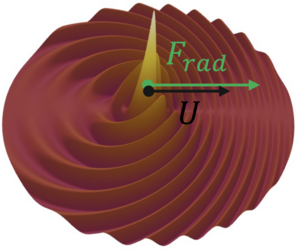
When an acoustic source starts translating, this translation induces an asymetry of the radiated acoustic field due to Doppler effect. Recently we have shown theoretically that this asymetry provokes a self-induced acoustic radiation force, which can either slow down the source (as in the case of a monopolar source) [A38] or accelerate it (in the case of a dipolar source) [A42]. In the latter case, the source is propulsed by its own wavefield, leading to an analogy with the De Broglie double-solution pilot-wave theory, similarly to what was unveiled by Couder & Fort for the hydrodynamic quantum analogues. We are now trying to materialize these "Acoustic quantum analogues" experimentally.
Cosmology analogues
In progress