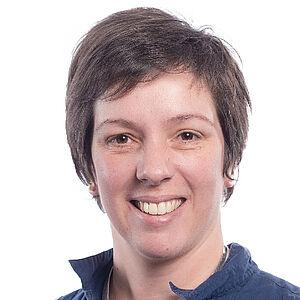
Valérie Vallet
Research activities
Methodological developments and modelling tools
Developments of classical force-fields
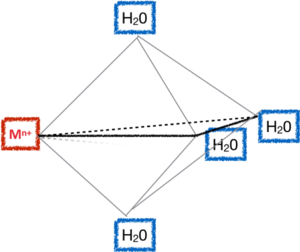
Depending on the system of interest and the conditions of the study, the description of the various interactions among the species, so called force fields (FF) may need to be developed, i.e. finding the most suitable analytical formula to represent the various type of interactions. In our approaches the analytical formula as well as the derived parameters are adjusted on highly accurate QM structures and energies of reasonable size (dimer interaction, clusters). For our systems of interest, covering a wide range of context from atmospheric, astrophysics, to nuclear fuel recycling, the group has developed FF for neutral and charged species accounting for polarization effects (L. Hormain, M. Monnerville, C. Toubin, D. Duflot, B. Pouilly, S. Briquez, M. I. Bernal-Uruchurtu, and R. Hernández-Lamoneda, J. Chem. Phys. 142, 144310 (2015) [DOI:10.1063/1.4917028], F. Réal, A. S. P. Gomes, Y. O. Guerrero Martínez, T. Ayed, N. Galland, M. Masella, and V. Vallet, J. Chem. Phys. 144, 124513 (2016) [DOI:10.1063/1.4944613]) and many-body corrections when required (F. Réal, M. Trumm, B. Schimmelpfennig, M. Masella, and V. Vallet, J. Comput. Chem. 34, 707 (2013) [doi: 10.1002/jcc.23184]).
Relativistic correlated methods
We develop electronic structure approaches that describe relativistic as well as electron correlation effects for systems across the periodic table. In complement to methods based on solving the Dirac equation for the electrons (four-component and two-component methods) so that spin-orbit coupling is treated at the mean-field level, such as CC, EOM-CC, with Jean-Pierre Flament, we develop an method that treats spin-orbit coupling via a spin-orbit CI (SOCI) [V. Vallet, L. Maron, C. Teichteil, and J.-P. Flament, J. Chem. Phys. 113, 1391 (2000); DOI: 10.1063/1.481929] approach on the basis of many-electron wavefunctions obtained with approximate relativistic Hamiltonians, describing only scalar relativistic effects (one-component methods). These developments are made on the EPCISO code.
Quantum embedding
Applying relativistic CC methods to get energies and spectroscopic properties for complex systems is very costly. We develop an embedding method – frozen density embedding (FDE) (A. S. P. Gomes and C. R. Jacob, Annu. Rep. Prog. Chem., Sect. C: Phys. Chem. 108, 222 (2012)[doi:10.1039/C2PC90007F], A. S. P. Gomes, C. R. Jacob, and L. Visscher, Phys. Chem. Chem. Phys. 10, 5353 (2008).[doi:10.1039/B805739G], S. Höfener, A. S. P. Gomes, and L. Visscher, J. Chem. Phys. 136, 044104 (2012); [doi:10.1063/1.3675845],S. Höfener, A. S. P. Gomes, and L. Visscher, J. Chem. Phys. 139, 104106 (2013) [doi:10.1063/1.4820488], M. Olejniczak, R. Bast, and A. S. P. Gomes, Phys. Chem. Chem. Phys. 19, 8400 (2017)[doi:10.1039/C6CP08561J]) - that let us treat a small part of the system with relativistic CC and the rest with more approximate methods such as DFT, giving us a fully quantum mechanical description of the whole system at an affordable computational cost. These developments are done on the Dirac (http://diracprogram.org), Dalton (http://daltonprogram.org) et ADF (http://www.scm.com), codes, as well as on the PyADF scripting framework (C. R. Jacob, S. M. Beyhan, R. E. Bulo, A. S. P. Gomes, A. W. Götz, K. Kiewisch, J. Sikkema, and L. Visscher, J. Comput. Chem. 32, 2328 (2011) [doi:10.1002/jcc.21810]), which let us combine different codes.
We have simulated the photoelectron spectra of halogens in water droplets with CC-in-DFT embedding (Y. Bouchafra, A. Shee, F. Réal, V. Vallet, and A. S. P. Gomes, Phys. Rev. Lett. 121, 266001 (2018) [doi : 10.1103/PhysRevLett.121.266001]) over structures from classical MD simulations with polarizable force fields (F. Réal, A. S. P. Gomes, Y. O. Guerrero Martínez, T. Ayed, N. Galland, M. Masella, and V. Vallet, J. Chem. Phys. 144, 124513 (2016) [doi:10.1063/1.4944613]).
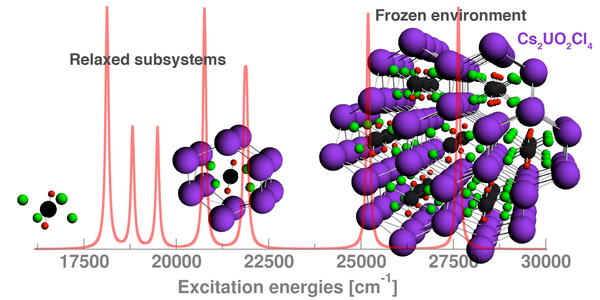
Molecular modelling of heavy elements
Modelling in bulk solutions and at interfaces
Our group develops polarizable force-field models (including many-body effects, such as charge-transfer, hydrogen bonding, etc.) to characterize the dynamics by classical molecular dynamics (Polaris(MD) package http://biodev.cea.fr/polaris/) of radioelements in bulk solution or at interfaces (organic/inorganic) (F. Réal, A. S. P. Gomes, Y. O. Guerrero Martínez, T. Ayed, N. Galland, M. Masella, and V. Vallet, J. Chem. Phys. 144, 124513 (2016) [doi:10.1063/1.4944613], F. Réal, M. Trumm, B. Schim- melpfennig, M. Masella, and V. Vallet, J. Comput. Chem. 34, 707 (2013) [DOI:10.1002/jcc.23184]). This work of relevance for medicine and the nuclear cycle (liquid/liquid separation processes) involves our partners from CEA Saclay (M. Masella, DSV), CEA Marcoule (E. Acher, D. Guillaumont, Lab LILA).
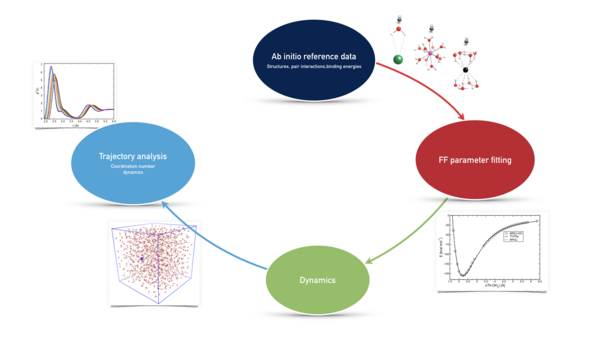
Radionuclides and nuclear safety
The predictive modeling of the nature and amounts of radioactive, such as Ru or Pu oxides species that might be released in volatile forms in case of a nuclear accidental is of key importance for nuclear safety agencies and risk analysis. Our group uses highly accurate quantum chemical methods (multi-reference ones, if needed, eg. CASSCF, CASPT2, DMRG), and developed in the PCMT team (EOM-CCSD), to provide reliable and accurate chemical and thermodynamic properties of gaseous radioactive species (eg. F. Miradji, F. Virot, S. Souvi, L. Cantrel, F. Louis, and V. Vallet, J. Phys. Chem. A 120, 606 (2016)[DOI: 10.1021/acs.jpca.5b11142])
These research activities are supported by the Excellence Lab CaPPA, and pursued in cooperation with the French Institute for Radioprotection and Nuclear Safety, IRSN.
Spectroscopic properties
We use electronic structure methods different degrees of accuracy (EOM-CCSD, CASPT2, DFT, …) to characterize how molecular species interact with electromagnetic radiation, which is the basis for different spectroscopic approaches (IR/Raman, UV-Vis, Fluorescence, XAS, XPS, NMR,…). These studies are performed for species the gas phase or in condensed phase (where we combine different methods via quantum embedding approaches). Our aim is to provide models that can help us connect experimental observation to physical processes occurring at the nanoscopic scale (A. S. P. Gomes, C. R. Jacob, F. Réal, L. Visscher, and V. Vallet, Phys. Chem. Chem. Phys. 15, 15153 (2013) [doi: 10.1039/C3CP52090K] ; P. Lindqvist-Reis, F. Réal, R. Janicki, and V. Vallet, Inorg. Chem. 57, 10111 (2018) [doi: 10.1021/acs.inorgchem.8b01224]).
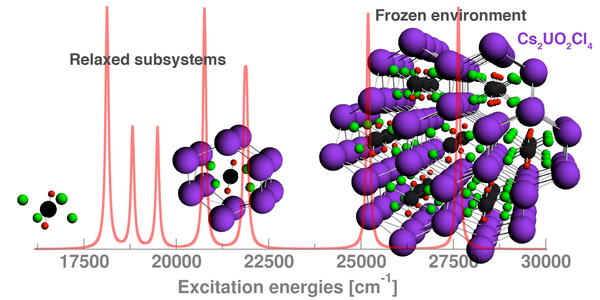